We do fundamental, curiosity driven research in soft matter physics. You can find out more about current topics in the short descriptions below, or on the Publications page.
Contact Electrification
Contact electrification (CE), also known as “tribocharging” or “triboelectrification”, is the exchange of electrical charge between materials during contact. Although ubiquitous in daily life (commonly conflated/confused with “static electricity”) and described even as far back as ancient Greece, we know surprisingly little about this effect. For metals, it is known that CE depends on the work function—the same parameter involved in the photoelectric effect. For most insulators, this parameter isn’t easily defined or obviously relevant, yet these materials charge more than metals. Understanding CE is extremely important as it plays a critical role in both technological and natural settings. For example, tribocharging may be the key ingredient that helps tiny dust particles in protoplanetary disks aggregate into ever larger and larger bodies in the millimeter to meter range, thus helping rocky planets such as the earth to form.
A long-term goal of our lab is to understand how tribocharging occurs for insulating materials. Previous models proposed that trapped electrons—those caused by material defects—might be the culprit, but thermoluminescence data suggest that the effect can occur when these are not present (see articles in Physical Review Letters and Nature Physics). More recent hypotheses suggest that different mechanisms may be at play for different classes of materials, separating mechanochemical effects for soft and amorphous polymers from adsorbate-driven effects with hard inorganic insulators. We are forging ahead in the development of an array of techniques to test these hypotheses. For the latest results, check out our Publications.
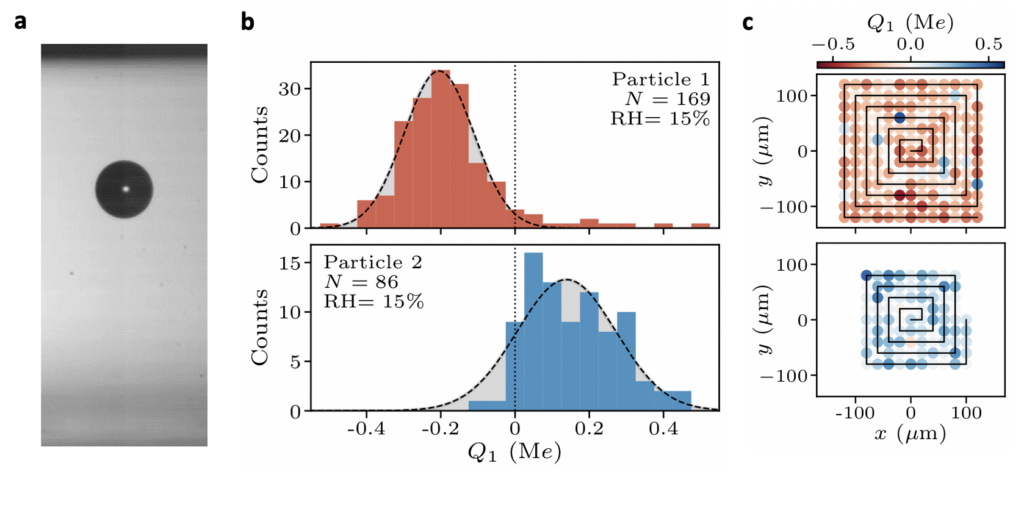
The Leidenfrost Effect with Soft and Vaporizeable Solids
In the “traditional” Leidenfrost effect, a liquid droplet placed above a surface whose temperature is far above its boiling point counterintuitively doesn’t boil, but rather gently glides over the surface. This occurs because the droplet vaporizes rapidly enough on its underside to levitate on its own vapor. While long known with liquids, we have discovered a new type of Leidenfrost effect that occurs with vaporizable soft solids, e.g. hydrogels (see our article in Nature Physics). Rather than hovering, these materials spontaneously start bouncing on a hot surface. This effect is dramatic—a 1 cm hydrogel sphere easily bounces to 5 cm heights for several minutes. The mechanism comprises an unanticipated new way to extract mechanical energy, with the sphere acting as a “soft engine” and siphoning energy from the steam it creates. We are carrying out experiments to understand this new type of Leidenfrost effect more thoroughly. Currently we are focusing on the transition to between the floating and bouncing regimes. To find out more about what we’ve already done, check out the papers in (Nature Physics, Physical Review Letters, Soft Matter), or read this nice news article in The Washington Post.
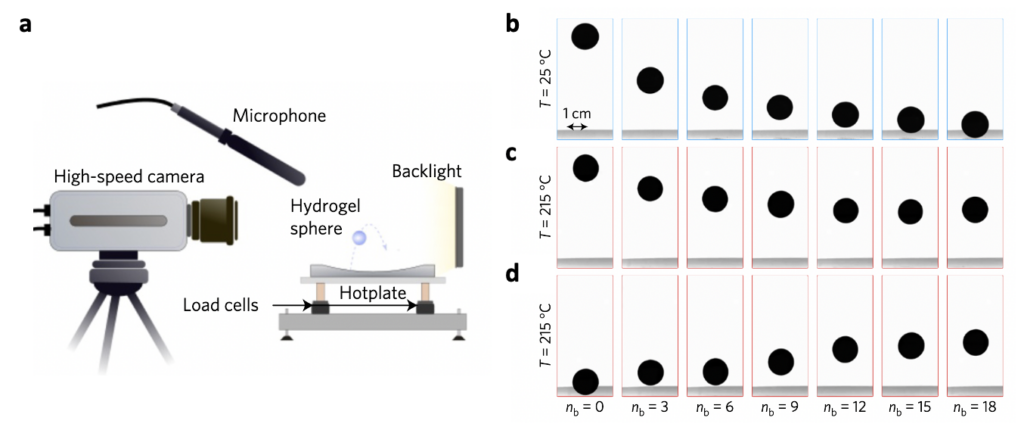
Active Matter with Quincke Rollers
One of the cornerstones of physics is the ability to describe the behavior of large systems of particles through statistical means. For example, to understand the behavior of molecules of gas in a room, it is impossible to know the position/velocity of every particle, but using a statistical analysis many useful laws can still be obtained (e.g. the ideal gas law). This “statistical mechanics” relies on the fact that such systems are in equilibrium, i.e. they conserve energy and have already evolved to their most probable state. For the past 20 or so years, a big push in physics has been to understand similar ideas in non-equilibrium systems, in particular active matter. In active matter systems, the particles are self-propelled, meaning they constantly absorb energy from some external source to force them into motion. This is different from the molecules in a gas, which are passive and only bounce around and redistribute energy like “billiard balls.” We do active matter experiments using “Quincke rollers.” In this work, small (~5 micron) particles are placed on a substrate in a slightly conducting fluid. When an electric field is applied through the fluid, the particles spontaneously begin to roll around the substrate. Our current interest is to find ways to control the motion of these spheres as they roll about, hopefully leading to the potential for controlling them at the individual level.
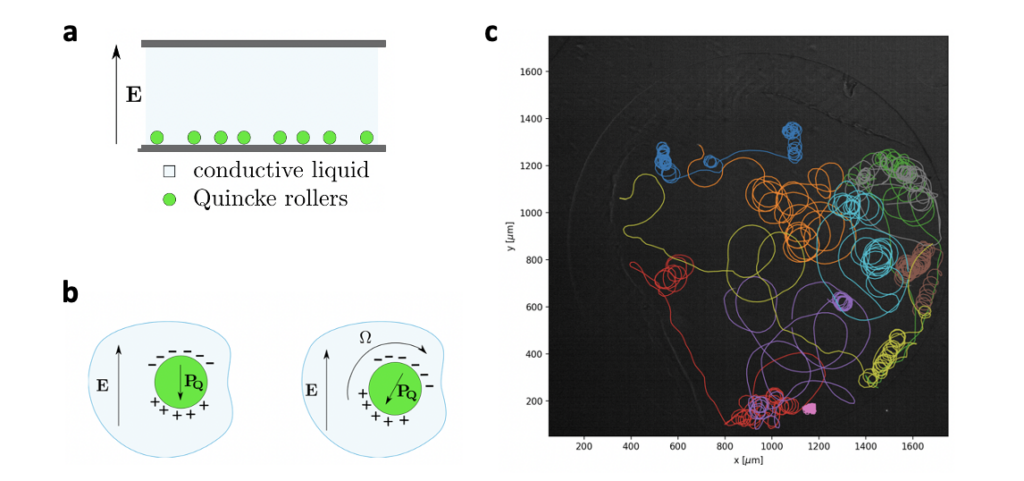